Pollution
4.3 Proper Management of Phosphorus for Future Food Security
Alyssa M. Zearley
Phosphorus is an important nutrient in fertilizers used in agricultural systems. Excess phosphorus from field runoff, storm water, and wastewater has led to harmful algal blooms in rivers, lakes, and other bodies of water. What are methods to curb phosphorus related pollution? Phosphorus is also quickly becoming a scarce resource worldwide. With an increasing global demand and reserves dwindling, will alternative sources of phosphorus be discovered?
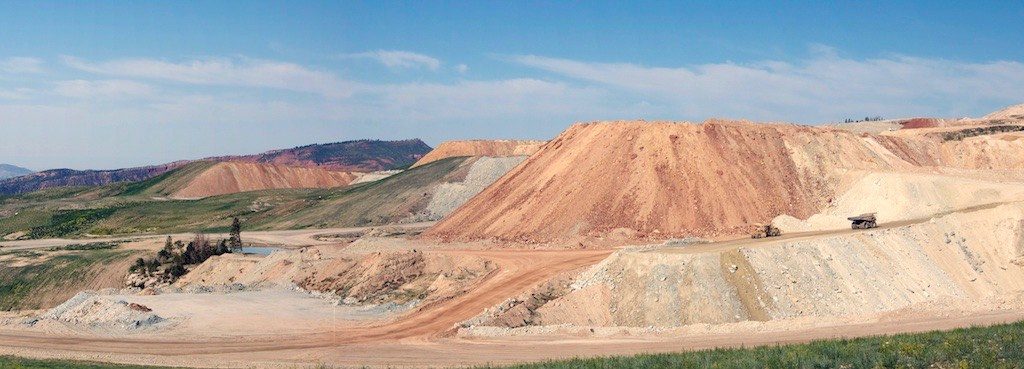
Photograph by Jason Parker-Burlingham, 2008. CC BY 2.0.
Phosphorous is an essential plant nutrient. Along with nitrogen (N) and potassium (K), phosphorus (P) is one of the three plant macronutrients. Phosphorus is used by plants in seed and fruit production, energy transfer, root growth, and maturation. A deficiency in phosphorus can lead to small fruit, reduced plant growth, and delayed maturity. Phosphorus is also critical for growth and development in animals. Animals use phosphorus in assimilating DNA, development of skeletal tissue, and for energy transferences. Understanding the phosphorus cycle is necessary to see how phosphorus moves through an ecosystem. Inorganic phosphorus is released by rock erosion and plants take up this phosphorus and transform it into organic phosphorus. Animals gain organic phosphorous by consuming plants or other animals. Phosphorus is returned to the soil by animal excretion or decomposition, and then it is either absorbed by plants or leached into waterways (Figure 2). Humans have altered the phosphorus cycle by adding phosphorus for crop production and removing phosphorus through soil management practices, which leads to erosion and leaching. Human manipulation of the phosphorus cycle negatively impacts the environment and future food production by removing phosphorus from ecosystems.
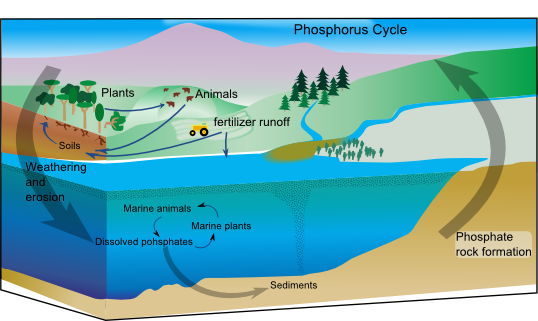
Courtesy of Bonniemf Incorporates work by NASA Earth Science Enterprise, 2013. CC BY-SA 3.0.
The first phosphorus fertilizer was manure, which has been used to enrich soil since animals were domesticated. Synthetic, inorganic phosphorus fertilizers were introduced in the mid 1800’s. As the technology improved, synthetic fertilizers increased crop yields and were less expensive than alternatives. While the application of manure from on-site farm animals did not significantly alter the phosphorus cycle, the addition of synthetic fertilizers increased phosphorus levels in ecosystems. Annual cropping systems result in the majority of the plant being removed after harvest, instead of decomposing and returning nutrients to the soil. However, the largest source of phosphorus loss derives from soil management practices that lead to leaching. Improper manure or fertilizer application, broadcasting fertilizers, no-till production, and erosion can cause phosphorus to leach into runoff, which then enters the waterways.1,2,3 This excess phosphorus travels from the soil to aquatic ecosystems in which phosphorus is a limiting nutrient. The introduction of phosphorus from synthetic fertilizers, excess manure, and sewage effluent contribute to eutrophication, which triggers algal blooms (Figure 3). Excess phosphorus creates ideal conditions for algae to flourish. Many algae produce toxins that are harmful to both humans and wildlife.4 Algal blooms also deplete oxygen in waterways and suffocate other organisms in a phenomenon known as hypoxia.5
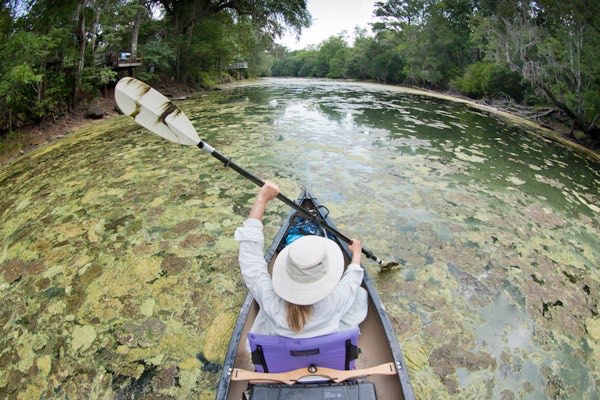
Photograph by John Moran, 2012. Public Domain.
In addition to the negative effects associated with excess phosphorus, the production of synthetic fertilizers also has adverse environmental impacts. Phosphorus mining is the fifth largest mining industry in the United States, with the largest mines in Florida, North Carolina, Tennessee, and Idaho (Figure 1). There are two processes for extracting phosphorus from ore: the wet-acid process and the thermal process. The wet-acid process creates calcium sulfate as a byproduct, which is known as phosphogypsum. This material has no practical uses and it is stored in enormous stacks which can cover several hectares and be nearly 60 meters high. Phosphate slag is the waste product created from the thermal process, and it can be used in construction, but much of it is also stored in stacks. The production process concentrates the radioactive isotopes naturally found in phosphate ores, and as a result the waste materials often have high concentrations of radium, uranium, and thorium.
Phosphogypsum is a byproduct of the wet-acid method of producing fertilizer from phosphate rock:
Ca5(PO4)3X + 5 H2SO4 + 10 H2O →
3 H3PO4 + 5 CaSO4 · 2 H2O + HX
where X may include OH, F, Cl, or Br
Due to its weak radioactivity, phosphogypsum is considered a waste product and is placed into storage indefinitely.
Phosphorus is a nonrenewable and finite resource, yet the global agricultural industry depends on phosphorus fertilizers to improve crop yields. It has been estimated that 30-50% of yields can be directly attributed to phosphorus fertilizers, and it would take only a small drop in production to cause major problems in the food system.
Phosphorus is becoming less abundant and more expensive as global demand increases. While phosphorus is found throughout the earth’s crust, it is not economical to extract it from anything but phosphate rock, which is a considerably rarer resource. Ninety percent of phosphate rock reserves are concentrated in just five countries: Morocco, South Africa, Jordan, China, and the United States.6 These reserves have been depleted by the extraction of the high quality ores. Increased production costs associated with extraction of inferior ores has caused fertilizer prices to rise. Reserves may become completely exhausted within 50-100 years. Some estimates put peak phosphorus, the point at which production peaks, as early as 2030.6 Demand for phosphorus and other fertilizers will increase tremendously as the world struggles to feed an increasing population.
To meet the demands of a burgeoning population, the phosphorus cycle will have to be closed. Once phosphorus reaches an ocean, it is essentially lost (Figure 2). It often takes millions of years for phosphorus deposited into ocean sediment to return to the surface.7 Phosphorus inputs must be decreased, maintained in the soil, and recaptured. Proper manure applications and soil management practices that decrease erosion can lessen the need for synthetic fertilizers. Increasing organic matter in agricultural soils decreases runoff and provides phosphorus to plants. Homeowners can reduce their phosphorus pollution by using products such as lawn fertilizers and detergents that do not contain phosphorus.
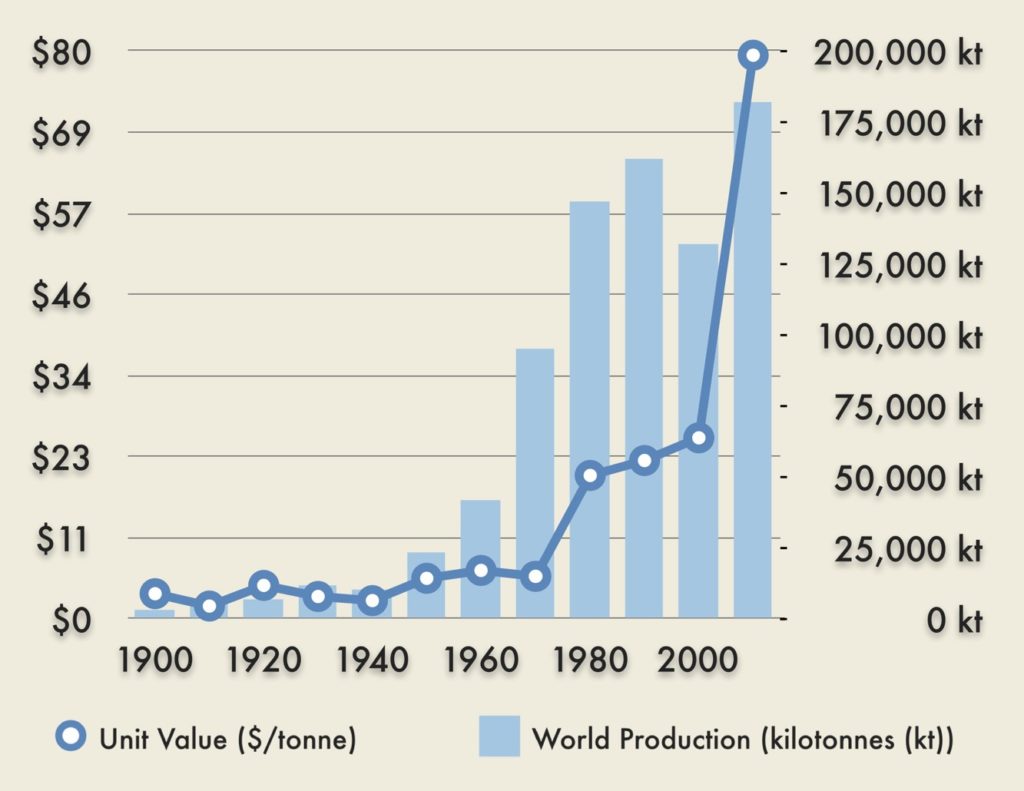
Data from U.S. Geological Survey, 2014. Public Domain.
There is ongoing research to discover solutions to the phosphorus problem. Alternative sources of phosphorus, such as animal carcasses, algae grown on animal manure, and sewage sludge have proved to be promising alternatives to phosphate rock.8,9,10 Utilizing these methods for phosphorus production would also provide alternate solutions for the pollution problems caused by these commonly unusable wastes. Although these research efforts are promising, countries are unable to recognize the impending phosphorus shortage. The efforts to find alternative phosphorus sources must be vastly increased to protect the environment and ensure sufficient phosphorus to feed future generations.
References
- Kleinman, P. J. et al. (2002). Journal of Environmental Quality, 31:2026-2033.
- Eghball, B., & Gilley, J. E. (1999). Journal of Environmental Quality, 28(4):1201-1210. doi:10.2134/jeq1999.00472425002800040022x
- McDowell, L. L., & McGregor, K. C. (1980). Transactions of the ASAE, 23(3): 643-648.
- Pitois, S., et al. (2001). Journal of Environmental Health, 64(5): 25-33
- Michalaka, A. M., et al. (2013). Proceedings of the National Academy of Sciences of the United States of America, 110(16):6448-6452.
- Cordell, D., et al. (2008). Global Environmental Change, 19:292-305.
- Paytan, A & McLaughlin, K. (2007). Chemical Reviews, 107(2): 563-576.
- Ma, Y. L. & Matsunaka, T. (2013). Soil Science and Plant Nutrition, 59(4): 628:641
- Mulbry et al. (2006). Journal of Vegetable Science, 12:107-125
- Gambus, F. (1998) Acta AGraria et Silvestria/ Agraria, 36:23-35.
- Parker-Burlingham, Jason. (2008). [Photograph of Phosphate Mine near Flaming Gorge NRA, UT]. Retrieved from Wikimedia Commons. CC BY 2.0.
- Bonniemf Incorporates work by NASA Earth Science Entersprise. (2013). [Diagram of the Phosphorus Cycle]. Retireved from Wikimedia Commons. CC BY-SA 3.0.
- Moran, John. (2012). [Photograph of a canoeist’s paddle scooping up algae on Santa Fe River]. Retrieved from Wikimedia Commons. Public Domain.
- U.S. Geological Survey. (2014). Phosphate Rock Statistics. Retrieved from http://minerals.usgs.gov/minerals/pubs/historical-statistics/ds140-phosp.pdf